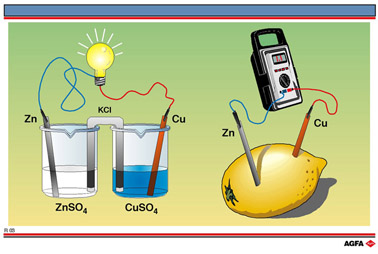
Aim: To demonstrate
the classic electrochemical cell and compare it with an unusual but analogous example |
In
illustration R2, it was shown that the electron transfer between I- and
iron(III) ions (Fe3+) can be used to produce an electric current by placing
platinum electrodes in the two solutions, connecting the electrodes with a wire and
connecting the solutions with a salt bridge. The two half-reactions, which make up the
electron transfer reaction, take place in the solutions in the two beakers.
This is
an electrochemical cell, and the beakers in which the two half-reactions take place are
its half-cells. An electrochemical cell is therefore a device which allows an electron
transfer reaction to take place in such a way that a flow of electrons is produced between
the electrodes.
If a
piece of zinc metal is placed in a blue aqueous solution of copper(II) sulphate (CuSO4)
then the colour intensity spontaneously decreases and the zinc takes on a red-brown
colour. An electron transfer takes place between the zinc metal and the copper(II) ions
with copper being deposited on the zinc metal, the solution becoming colourless as the
copper(II) ions are reduced on the zinc metal to copper and the zinc metal is oxidised to
colourless zinc ions. This reaction can be represented as:

This electron
transfer can, in principle, be observed indirectly by an increase in temperature
as the electron transfer energy is changed to heat energy.
This redox reaction can be broken down into the following half-reaction equations :
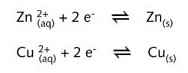
If these half-reactions
are set-up in separate beakers as in illustration R2 (right-hand side),
each containing the metals in the form of electrodes immersed in an aqueous
solution of the corresponding metal salt and with the solution connected
by a salt bridge and the metal electrodes linked by wires to a lamp as
shown in illustration R3, we again create a source of electric current.
This demonstrates
the involvement of electron transfer.
If this simple electrochemical cell is kept at a temperature of 25°C (298K)
and the concentrations of the zinc and copper ions are both 1mol/L, then
we obtain what is known as the Daniell cell.
The tendency of electrons to flow through the external circuit of a cell
is quantified as the e.m.f. , the electromotive force of a cell, also
called the cell potential Ecell.
The e.m.f of a cell can be measured using a high resistance voltmeter,
which takes negligible current from the cell.
In the case of the Daniell cell the Ecell is +1.1 V.
The cell potential is by convention taken to be acting from left to right
through the cell with the (more) negative electrode on the left.
Thus

The cell can be represented
as :

|
|
Electrodes are represented according to IUPAC
as solid vertical lines I, the salt bridge by double vertical dashedlines. In practice sulphate salts are used
in this cell although nitrate, chloride or hydrogen sulphate salts could be used.
The salt bridge, as also shown in illustration R2, contains KCl or KNO3. It is
a sufficiently good conductor of ions to produce good contact between the solutions.
It does not contain possibly interfering species.
When the electrodes are connected, current begins to flow and the reaction starts. As the
electrons flow between the zinc and copper electrodes the concentration of zinc ions
increases in one half-cell while the concentration of copper(II) ions decreases in the
other half-cell leading to an ionic imbalance.
This results in a decrease in the potential difference between the two half-cells. The
salt bridge between the two solutions enables an ionic balance to be maintained without
the transport of ions from one half-cell solution to the other. The positive ions
(cations) of the salt bridge diffuse to the sulphate solution and the negative ions
(anions) to the solution of zinc ions.
The reaction proceeds and the potential difference between the electrodes decreases
eventually reaching zero when either all the copper(II) ions have or the zinc electrode
has been totally consumed.
On the right-hand side of illustration R3 a lemon is shown with two electrodes stuck in
it. One is zinc and one is copper. If these electrodes are joined by a wire to a voltmeter
then a voltage (admittedly lower than 1.1 V) can be measured. Again the zinc is
functioning as the anode and the copper as the cathode.
The lemon must contain ions
which function in the same way as the Cu2+ and Zn2+ ions in the
Daniell cell. The diffusion of the ions is slow and so the voltage is lower but is
measurable, even though both electrodes are in one solution without a salt bridge.
Cell
electrodes
A metal consists of cations of the metal (positively charged ions) surrounded by
electrons. If a strip of metal is placed in a solution of its ions some of the cations in
the metal may dissolve leaving a build up of electrons on the metal:

The metal strip
will become negatively charged.
Alternatively metal ions in the solution may take electrons from the strip of metal and be
discharged as metal atoms:
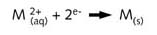
The potential
difference between the strip of metal and the solution depends on the
nature of the metal and the concentration of the ions involved in the
equilibrium at the metals surface. If we compare zinc (Zn) and copper
(Cu) for example, then, for the same concentration of ions in each solution,
zinc acquires a more negative potential than copper since it has a greater
tendency to dissolve ions and a smaller tendency to be deposited as a
metal. Hence in such a system as is shown in illustration R3 the zinc
electrode is the anode and the copper electrode the cathode.
At the anode oxidation takes place : here zinc metal is converted into zinc ions.
At the cathode reduction takes place : copper(II) ions are reduced to copper metal.
In galvanic cells, the anode is indicated by the negative sign s and the cathode by the sign r.
In electrolytic cells (see R11) an anode, being the electrode where oxidation takes place,
gets the positive sign r. A cathode of an electrolytic cell is
indicated by the negative sign s.
For a galvanic cell, the cell voltage or cell potential, in terms of anode and cathode is
written as :

|